- Adi Thakur
- Jul 4, 2021
- 7 min read
The Moons of Saturn have proven to be an area of research with constant scientific interest. Rhea, one of the Moons existing in this system, is no ordinary astronomical body. From its size, the second largest Moon of Saturn, to its name, borrowed from Greek Mythology, Rhea signifies a body of aura and mystery in equal proportions.
Rhea was first discovered in 1672 by Giovanni Cassini whose achievements are of such magnitude that he possesses astronomical discoveries (Cassini Division) and space probes (Cassini Space Probe) as acknowledgments for his phenomenal work in astronomy. The body was the second satellite of Saturn discovered and the third object found to be orbiting the planet. Cassini had named this body, along with four other satellites of Saturn, “The Stars of Louis.” It was only in 1847 that English astronomer John Herschel suggested the name Rhea.
The early 21st century has proven to be pathbreaking in the study of Rhea. While the original hypothesis suggested that Rhea consisted of a rocky core, analysis of data from the Cassini flyby mission of 2005 proved otherwise. From these data, astronomers found the value for the standard gravitational parameter (GM), which is the product of the gravitational constant and the mass of the body, to be 153.93950.0018 km3s-2. From this, the density of Rhea was calculated to be 1232.85.4 kgm-3. Results for J2 and C22, degree-2 gravity coefficients, were found to be (7.9470.892)10-4 and (2.35260.0476)10-4. The ratio of these values suggests that Rhea has a hydrostatic equilibrium, which describes a balance between gravitational force, which works to contract an object, and pressure from the core, which works to expand an object. This equilibrium allows the body to maintain a spherical shape with no deviations from sphericity.
These gravity coefficients, along with the newfound conclusion that the body has a hydrostatic equilibrium, have also allowed the calculation of the moment of inertia, which is the radial distribution of mass in the satellite, using the formula: C/MR2to be 0.37210.0036 or approximately 0.4 which, theoretically, is characteristic of a spherical body with uniform density. (Iess et al.)
Through these analyses and calculations, astronomers continued to build sophisticated models for the interiors of Rhea. Most of such models describe an undifferentiated, or only weakly differentiated, satellite. These models have been backed by data from the flyby missions as well as the fact that the C22value is greater than the amount required for non-differentiated interiors to be existing (Castillo-Rogez.) The interior is further estimated to be composed of 25% rock and 75% ice mixtures.
These insights have been challenged over time. A paper from 2007 challenges the findings of a hydrostatic equilibrium and that the body is undifferentiated. By carrying out thermophysical dynamical evolution models of Rhea, this paper proposed that differentiation must have occurred near the origin of the body. The authors further go on to use their model to provide explanations of some non-hydrostatic anomalies that were observed by the Cassini mission. The paper suggests that the hypothesis of hydrostatic equilibrium was accepted a priori and therefore affected the results. Updated estimates for the gravity coefficients are also provided to back their claims. (Mackenzie et al.)
However, a different paper published in 2008 refuted these claims. The author of this paper suggests that the flyby mission returned “rather useless results” which gave no indication of the interior structure of Rhea. He goes on to describe how data from Doppler studies indicated that there was no convincing evidence that Rhea is nonhydrostatic (Anderson). Thus, the hypothesis of hydrostatic equilibrium was not rejected and the compositional makeup of the body (rock & ice) was found.
Emerging from the interior, the surface of Rhea, as is true for all the Moons of Saturn, is heavily cratered. Additionally, the planet is characterised by two large impact basins: Tirawa, which is upto 5 km deep and 360 km across, and Inktomi, with characteristics as interesting as its name. Known by its nickname ‘The Splat’, the basin is 47.2 km across and the impact that created it supposedly has ice from beneath Rhea’s surface. This phenomenon has resulted in the region appearing lighter than its surroundings. This light makes it extremely apparent in any image of Rhea, and is shown in Figure 1. Additionally, the impact also resulted in an ejecta blanket, which is a ring of ejecta surrounding a crater. Due to this observation, astronomers believe this to be a recent impact event.
Figure 1
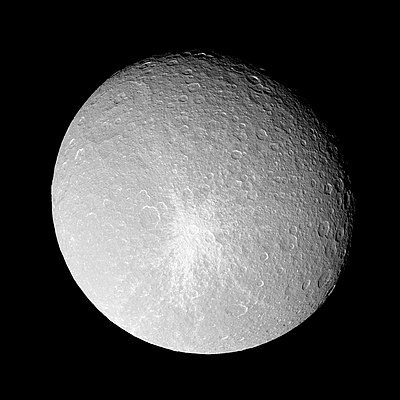
What makes this Moon all the more intriguing is the fact that it may be the first of its kind to possess a ring system. This system is hypothesized to contain three dense, narrow bands within a disk surrounding the body.
In 2005, during Cassini’s flyby mission, the spacecraft had collected data from the plasma shadows (the regions where plasma from the magnetosphere of Saturn was blocked by the Moon) of Moons such as Dione and Tethys. The observations revealed an abrupt drop in energetic electrons, caused mainly by the blocking of the Moon. In Rhea’s observations however, the spacecraft found startling results.
There was a gradual decrease in the presence of energetic electrons in the distance leading up to the plasma shadow of Rhea. When the spacecraft entered the shadow, the expected cutoff was observed. But this distance over which electrons seemed to be decreasing in presence intrigued astronomers. Moreover, as the spacecraft exited from the shadow, a gradual increase in electrons was noticed, indicating a symmetric pattern of behaviour. To add to this symmetry, three sharp drops in electron presence were detected prior to entry and after exit from the plasma shadow. Further, the distance over which a gradual decrease and later increase of electrons was observed was corresponding to the Rhea’s hill sphere (the region around a body where any satellites are attracted into their orbit).
While initial study prompted gas and dust particles in the magnetosphere to be the likely candidates of such decreases, the spacecraft was not able to find these particles in large amounts. Therefore, the focus turned to larger, solid particles upto 1min size. These particles are predicted to have ‘soaked up’ the electrons forming a disk. This disk is said to be denser nearer to the Moon, which caused the drop in electrons, with the explanation for the three sharp anomalies being narrow disks of higher particle density (Hecht).
However, not all evidence supports this theory of a ring system. The primary source of objection is due to the fact that observations made by the Cassini ISS Narrow-Angle Camera failed to gather any evidence or material that can prove the existence of the ring system. A paper from 2010 rules out any solid objects or a disk-like structure to be the cause of the electron absorptions, essentially rejecting the hypothesis put forward by earlier studies (Tiscareno et al).
This paper further puts forward a theory that for any object to be absorbing these electrons, it must be four orders of magnitude larger than any particles observed currently. Specifically, this object must have an extinction coefficient, which can be written as r2nwhere r is the particle radius and n describes the density, more than four times that of any of the current observed particles.
The paper concludes by stating the codependent relationship between a particles’ mass and their abilities to absorb electrons. A large mistake made by earlier papers, according to the authors, was to consider the volume of a particle, rather than the mass, which would allow not just a ring of particles, but also clouds of matter around Rhea.
But, despite all this evidence, there’s an opening just wide enough for some astronomers to pursue this mystery further. Along the equator of Rhea, there are blue marks on the surface left by fresh blue ice which suggests an impact from deorbiting ring material. Figure 2 shows the blue marks on the surface.
Figure 2: Blue spots visible on the surface of Rhea, possibly from deorbiting ring material
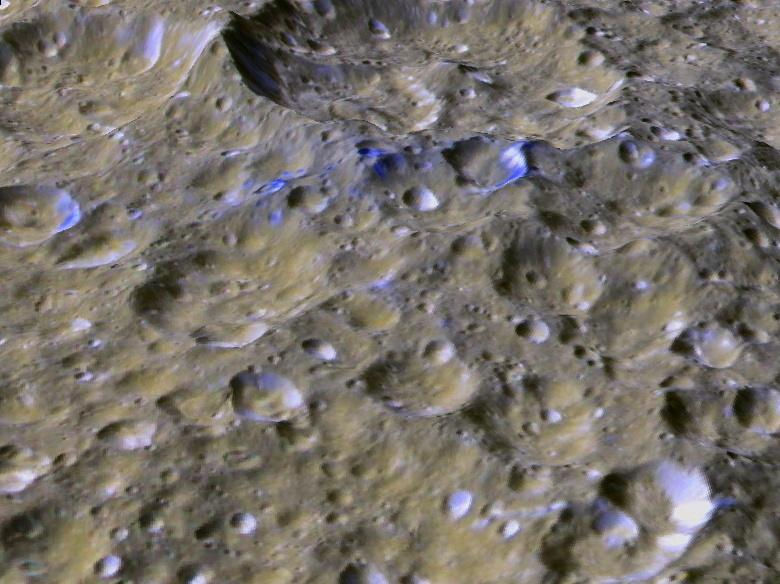
Despite many astronomers rejecting the hypothesis of Rhea containing a ring system, the electron depletion pattern remains a reality, the cause of which is still largely unknown.
Energetic particles, such as the electrons discussed prior, also cause a different phenomenon in the atmosphere of Rhea. Cassini first detected Rhea’s exosphere and found oxygen and carbon dioxide molecules in it. Oxygen molecules originate from the energetic particles in Saturn’s magnetosphere. The particles collide with Rhea’s surface when Saturn’s magnetic field rotates over Rhea and cause chemical reactions releasing oxygen molecules as a byproduct.
This was not only the first time a space mission found oxygen molecules in an atmosphere other than Earths, but also suggests active chemistry occurring within Rhea’s atmosphere, a key ingredient for life on the satellite (Cook).
Life on the Moon is still far from reality, however.
Rhea’s temperature can reach upto 99K while in direct sunlight and range between 73K to 53K while in shade. Therefore, it is too cold on the surface for human life. And although we have made progress by identifying oxygen in the exosphere, this still does not translate to liquid water until the oxygen molecules are transported to a subsurface ocean, where life can be formed. No evidence of an ocean on Rhea has been detected till date.
The origin of the carbon dioxide still remains a mystery at large. Though there are many speculative theories, some backed by chemical knowledge, the true origin of these molecules is yet to be found.
Rhea continues to be a fascination and mystery for all those that study it. Possibilities of subsurface oceans, its largely unknown beginnings and the unapparent and possibly hidden system of rings make this body one of the more unexplored and mysterious satellites in our Solar System. Any further studies, particularly ones attempting to find the cause behind the depletion of electrons in the magnetosphere of Saturn and its link to Rhea, are particularly interesting and, according to me, require and deserve more attention.